Επιστημονική Εργασία Ιατρού Ενδοκρινολόγου ΙΣ Κέρκυρας κ Ελένης Κούστα
05/02/2025
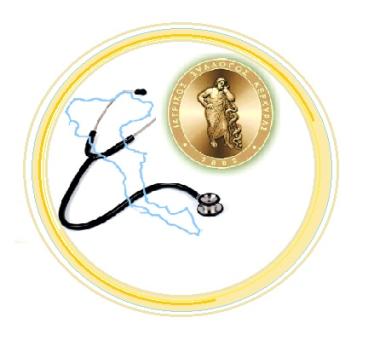
Citation: Chatzianagnosti, S.;
Dermitzakis, I.; Theotokis, P.; Kousta,
E.; Mastorakos, G.; Manthou, M.E.
Application of Mesenchymal Stem
Cells in Female Infertility Treatment:
Protocols and Preliminary Results.
Life 2024, 14, 1161. https://doi.org/
10.3390/life14091161
Academic Editor: Giuseppe Grande
Received: 22 July 2024
Revised: 28 August 2024
Accepted: 5 September 2024
Published: 13 September 2024
Copyright: © 2024 by the authors.
Licensee MDPI, Basel, Switzerland.
This article is an open access article
distributed under the terms and
conditions of the Creative Commons
Attribution (CC BY) license (https://
creativecommons.org/licenses/by/
4.0/).
life
Review
Application of Mesenchymal Stem Cells in Female Infertility
Treatment: Protocols and Preliminary Results
Sofia Chatzianagnosti 1 , Iasonas Dermitzakis 2,* , Paschalis Theotokis 2 , Eleni Kousta 1 ,
George Mastorakos 3 and Maria Eleni Manthou 2
1 School of Medicine, National and Kapodistrian University of Athens, 11527 Athens, Greece;
sofiachatzianagnosti@gmail.com (S.C.); lkousta@gmail.com (E.K.)
2 Department of Histology-Embryology, School of Medicine, Aristotle University of Thessaloniki,
54124 Thessaloniki, Greece; ptheotokis@auth.gr (P.T.); mmanthou@auth.gr (M.E.M.)
3 Department of Endocrinology, Diabetes Mellitus and Metabolism, Aretaieion Hospital, Medical School,
National and Kapodistrian University of Athens, 11527 Athens, Greece; mastorakg@gmail.com
* Correspondence: iasonasd@auth.gr
Abstract: Infertility is a global phenomenon that impacts people of both the male and the female sex;
it is related to multiple factors affecting an individual’s overall systemic health. Recently, investigators
have been using mesenchymal stem cell (MSC) therapy for female-fertility-related disorders such as
polycystic ovarian syndrome (PCOS), premature ovarian failure (POF), endometriosis, preeclampsia,
and Asherman syndrome (AS). Studies have shown promising results, indicating that MSCs can
enhance ovarian function and restore fertility for affected individuals. Due to their regenerative
effects and their participation in several paracrine pathways, MSCs can improve the fertility outcome.
However, their beneficial effects are dependent on the methodologies and materials used from
isolation to reimplantation. In this review, we provide an overview of the protocols and methods
used in applications of MSCs. Moreover, we summarize the findings of published preclinical studies
on infertility treatments and discuss the multiple properties of these studies, depending on the
isolation source of the MSCs used.
Keywords: molecular techniques; mesenchymal stem cells; stem cell therapy; signaling pathways;
reproductive system; infertility treatment
1. Introduction
Infertility is characterized by the inability to achieve pregnancy, while trying to, for
at least 12 months, including the occurrence of miscarriages [1,2]. This condition affects
48 million reproductive-age couples globally, with men and women each contributing 40%
of cases, while the remaining 20% are due to both partners or unexplained causes [3,4].
More specifically, the most common causes of subfertility include the following: male factor
subfertility (30%), such as defects during spermatogenesis; ovulatory dysfunction (25%),
such as polycystic ovary syndrome (PCOS); hypothalamic dysfunction, primary ovarian
insufficiency (POI), or tubal issues (20%); uterine or peritoneal disorders (10%) [1,5–7].
Age-related factors, infections, and environmental and lifestyle factors such as smoking or
obesity may also contribute to subfertility. Additionally, medical treatments like surgery,
radiotherapy, or chemotherapy for severe diseases such as cancer can compromise fertility
[8–13].
Numerous molecular factors that are involved in these reproductive disorders reflect
an individual’s overall systemic health, to the same extent as the complexity of related
signaling pathways [14–16]. The lack of specific biomarkers and knowledge of the mechanisms
involved in these conditions increases the gap between successful diagnoses and
effective treatments, indicating a need for extended research.
Life 2024, 14, 1161. https://doi.org/10.3390/life14091161 https://www.mdpi.com/journal/life
Life 2024, 14, 1161 2 of 21
Currently, stem cell therapy, particularly mesenchymal stem cell (MSC) therapy, is
emerging as a promising treatment for subfertility conditions such as PCOS, POI, endometriosis,
Asherman syndrome, and anovulation after cancer treatment. The ability
of MSCs to self-renew and differentiate into multiple cell types, along with their antiinflammatory
and proangiogenic effects, make them potential candidates for enhancing
pregnancy outcomes [17–22]. However, extensive research is still required to fully understand
stem cell biology and optimize clinical applications.
In this review, we discuss the current status, existing biological mechanisms, and
clinical applications of MSC therapies in patients facing infertility-related issues. We
mention their properties, which emerge from their source of isolation; additionally, we
discuss the protocols, methods, and molecular techniques employed for MSCs’ applications
in clinical practice. This information may be useful to investigators working on MSC
therapies for restoring fertility.
2. Stem Cell Therapy and the Role of MSCs
So far, people wishing to conceive who are facing infertility issues will either receive
medication, undergo surgical procedures, or undergo cryopreservation treatments in combination
with assisted reproduction technologies in an effort to achieve a positive pregnancy
outcome [3,14,23,24]. However, many complications, such as multifetal gestations, ectopic
pregnancy, ovarian hyperstimulation syndrome, ischemic injury limitations, birth defects,
high risk of breast and ovarian cancer, and vein thromboembolism, are associated with
the therapeutic methods discussed in [3,8,14,24]. Investigators have indicated that the
overall infertility rate remains greater than 80% [14,25]. Developing an effective therapeutic
approach requires significant consideration of physical, psychological, financial, and
time-related factors. Additionally, it is essential to utilize new cellular-level technologies to
aid in building a full understanding of underlying molecular mechanisms [14].
Stem cells are defined as cells which remain in an undifferentiated form in embryos
and adult tissues and which have a self-renewal ability and a differentiation ability in
multiple mature cell types under certain conditions [3,24,26,27]. Thus, they can contribute
to an organ’s damage repair and restoration level. They are classified, according to their
origin, as embryonic stem cells (ESCs), adult stem cells (including mesenchymal stem
cells, MSCs), induced pluripotent stem cells (iPSCs), spermatogonial stem cells (SSCs),
and ovarian stem cells [24,28,29]. However, ESC therapies face ethical and controversial
concerns regarding potential tumor formation, lack of functionality, immune rejection,
and inefficiency after transplantation [3,6,30,31]. Moreover, their difficult and demanding
manipulation protocols, which include the handling of early-stage embryos, have led to
religious and political concerns, to the same extent as the induction of abortion. Therefore,
distinct policies and ethical regulations are necessary if they are to become therapeutically
applicable [6,32,33].
Mesenchymal stem cells (MSCs) have received increasing attention for their potential
applications in several diseases; this is particularly the case for fertility-related disorders,
as they can restore ovarian function [3,7,15] due to their easy in vitro isolation protocols
and culture handling, which have not led to major ethical concerns [7,8]. MSCs are spindleshaped
somatic cells with a stromal origin; they can be extracted from several sources,
such as bone marrow, adipose tissue, menstrual blood, umbilical cords, amniotic fluid,
placental tissue, the endometrium, and other tissues or organs [1,8,15,34]. Moreover, they
can modulate immune responses and proliferate, differentiate, self-renew, and interact
through cell–cell signaling; most importantly, they secrete paracrine growth factors, such as
chemokines, cytokines, miRNAs, and extracellular vesicles, resulting in tissue regeneration
and repair [6,14,24]. Specifically, with reference to infertility treatments, their most prominent
features include their ability to differentiate and their secretory, immunomodulatory,
and anti-inflammatory capacity; additionally, they can perform mitochondrial transfer
following migration to injured tissues [3].
Life 2024, 14, 1161 3 of 21
2.1. Protocols for Cultures of MSCs and Therapeutic Methods Employing MSCs
Mesenchymal stem cells can be categorized based on their source: bone marrow
MSCs—BM-MSCs; umbilical cord stem cells—UC-MSCs; amniotic fluid mesenchymal stem
cells—AF-MSCs; menstrual stem cells—Men-MSCs; adipose-derived stem cells—AD-MSCs;
placenta-derived stem cells—PMSCs [3,35]. They have been found to show efficacy in treating
fertility-related disorders in both preclinical and clinical studies [3]. Their effective
differentiation and proliferation levels are linked to various parameters, such as the age of a
donor, the culture conditions, the isolation methodology, the materials employed, the route
of cell expansion, the route of administration, the cell dosage, and the microenvironment of
the source from which a sample of MSCs has been extracted [8,36,37].
The Mesenchymal and Tissue Stem Cell Committee of the International Society for
Cellular Therapy introduced specific criteria for MSCs used in research and clinical practice
[7,15,38]. Firstly, MSCs should be plastic-adherent when preserved in standard cell
culture media and be able to differentiate, under certain conditions, into osteoblasts,
adipocytes, and chondroblasts [15,39]. Secondly, they express specific cell surface molecules,
CD73, CD90, and CD105; but they do not express certain hemopoietic markers, i.e., CD11b,
CD14, CD19, CD34, CD45, CD79a, and HLA-DR [7,40,41]. Despite the common criteria
established, there is no universal agreement on the optimal source and method for
MSC isolation, purification, culture, or the animal model that should be used for their
extraction [42–45].
Isolation and culture protocols vary depending on the source of MSCs and involve
specific methodologies that are tailored to each tissue type. Solid tissue sources like bone
marrow require density centrifugation or enzymatic digestion, while blood or fluid samples
undergo simpler centrifugation methods [46]. Adherence properties aid in MSC isolation,
while contamination with hematopoietic stem cells necessitates sorting techniques, such as
magnetic bead sorting or fluorescence-activated cell sorting (FACS) [46].
The in vitro culture of cells is an essential requirement in the expansion and multiplication
of the number of MSCs, enabling a sufficient quantity to be reached so they can be used
effectively in medical treatment. Culture conditions, including medium composition and
incubation parameters, influence proliferation and differentiation potential. For instance,
MSCs are typically cultured in Dulbecco’s Modified Eagle Medium (DMEM) or Minimum
Essential Medium (MEM), supplemented with fetal bovine serum (FBS) or human platelet
lysate. L-DMEM, DMEM-F12, and LG-DMEM are also being used [8,9,47–50], with most
methods containing glucose and ions like magnesium, calcium, potassium, sodium, and
phosphate, and amino acids [46,51]. While monolayer cultures are common due to their
cost-effectiveness, 3D static cultures and bioreactors offer enhanced cell proliferation and
differentiation capabilities, mimicking in vivo conditions with minimal stress [46,52–55].
These scaled-up cell manufacturing systems overcome conventional methods in terms of
minimized media exposure and manufacturing time, as well as anticontamination and
labor costs [37,56,57].
Quality control during MSC expansion adheres to Good Manufacturing Practices
(GMPs), ensuring standardized production. Growth factors such as fibroblast growth
factors (FGFs) and platelet-derived growth factor BB (PDGF-BB) may enhance cell yield
and viability [44,45]. Long-term culture approaches present certain challenges, such as
reduced potency and senescence, which are mitigated by hypoxic conditions [37,58].
Characterization techniques include flow cytometry for surface marker analysis, trilineage
differentiation assays, gene expression profiling, and functional assays, such as
colony-forming unit assays (CFU-Fs). Delivery methods vary based on the therapeutic
target in question, with intravenous, intra-arterial, or local injections and surgical implantations
being common approaches [38,59–63].
After the ex vivo isolation and expansion of MSCs, the next critical step for clinical
application is to effectively deliver fresh cells to the target site. Common delivery methods
include the following: intravenous injection for systemic delivery, which is often used
in treating inflammatory or autoimmune conditions; intra-arterial or local injection, for
Life 2024, 14, 1161 4 of 21
targeted delivery to specific organs or tissues; surgical implantation, in which MSCs are
embedded in scaffolds [46]. Scaffolds consist of either substances found in the body,
such as collagen [35], or synthetic substances; scaffolds can be considered to be vehicles
for tissue regeneration. They can mimic the native characteristics of tissue and provide
vasculogenesis, cell migration, and the attachment of the MSCs [46].
In order to manage the homing of the stem cells and understand their regenerative
ability, several bioactive materials are tagged to the cells, providing a way of tracking
them and guiding them in the right direction. PKH26, green fluorescent protein (GFP), Ffluorodeoxyglucose,
enhanced green fluorescent protein (EGFP), CM-Dil, DiIC fluorescence
dyes, molday ion rhodamine B, and iron oxide particles are the substances that are used
more frequently in immunohistochemistry and fluorescence microscopy [7,15,18–20,62–69].
Moreover, additional methods include magnetic resonance imaging (MRI), in which MSCs
are labelled with iron oxide nanoparticles and provide high-resolution images, bioluminescence
imaging (BLI) using luciferase expression, positron emission tomography
(PET), single-photon emission-computed tomography (SPECT), and computed tomography
(CT) [64–66,70–72].
Storage and transportation protocols are critical in maintaining MSC viability and
functionality. Cryopreservation in liquid nitrogen or short-term refrigeration with cryoprotectants
ensures cell stability during transit, though challenges like cell viability and
apoptosis rates remain [37,67,68]. Regarding the short-term storage of MSCs, the cells
can be preserved at 4 ◦C for 72 h before transplantation using cryoprotectant mediums
such as DMSO. However, it is important to consider that frozen transportation can lead
to a substantial reduction in viable cells and an increase in apoptotic and senescent cells.
For non-frozen transportation, temperature preservation at 37 ◦C and cell metabolism
maintenance is challenging. Additionally, non-frozen methods are inconvenient due to
packaging limitations for long-distance transport [37,67,73].
Lastly, regarding the long-term storage of MSCs, stem cell banking aims to store the
maximum number of samples cost-effectively. Cryovials and cryobags are available in
various sizes. It is crucial that stem cell banks are able to maintain quality control, ensuring
the high quantity and quality of cryopreserved samples [37,69,73].
2.2. Biology of MSCs
The therapeutic properties of MSCs in fertility-related diseases look very promising in
cell therapy treatments. However, controversies and concerns surrounding their outcomes
in clinical practice remain in the spotlight; this is because there is a lack of knowledge
on the function of MSCs at the cellular and molecular levels [6,8,14]. Thus, developing
an effective therapeutic approach requires cellular-level technologies to fully understand
the underlying molecular mechanisms. These mechanisms are described further on in
this paper.
Mesenchymal stem cells can regulate immune response, inflammation, angiogenesis,
and oxidative stress in disorders, causing infertility through mechanisms and signaling
pathways which alter cell differentiation or contribute to the secretion of several transcription
factors that are essential for tissue restoration and improvement (Figure 1) [1,7,15,24].
Through the NF-kB/Rap1 pathway (a telomere-associated protein), MSCs can regulate
immune responses and inflammation [7,15,74] and delay the pro-inflammatory function
of the target tissue by increasing Treg production in multiple ways [7,75]. Firstly, they can
modify the cytokine profile of the dendritic cells that are responsible for initiating every
antigen-specific immune response [7,75]; also, they can convert T cells to Treg through the
transformation of M1 macrophages into M2 macrophages, resulting in tissue repair and
healing [3,76,77]. Secondly, MSCs can reduce the levels of IL-6 and IL-1β that are secreted
from macrophages, thus increasing the number of Treg cells [7,75,78]. Furthermore, they
are able to limit inflammation by secreting multiple factors such as TGFβ, HGF, lipoxinA4,
TNFα, PGE2, IDO, and NO, thus leading to increased levels of Treg cells by upregulating
the transcription of FOXP3/CTLA4/GITR genes [7,79–82]. In addition, MSCs can preserve
Life 2024, 14, 1161 5 of 21
immune tolerance by suppressing the differentiation of Th17 through the inhibition of
IFNγ production and the alteration of the response of Th1 to Th2 [7,75,78]; moreover, they
can decrease immune rejection through the downregulation of the MHC-II reaction [35,83].
Figure 1. Simplified overview of female reproductive disorders (Asherman syndrome, polycystic
ovarian syndrome (PCOS), premature ovarian insufficiency (POI), and endometriosis) in the internal
reproductive female organs, as well as the healing effects of mesenchymal stem cells (MSCs).
The differentiation capacity of MSCs is also essential for restoring infertility. They
can differentiate into epithelial, stromal, and endothelial cells and enhance the recovery of
ovarian function [1,3,7]. However, the number of MSCs that can differentiate and be integrated
functionally is too small, inhibiting observation of significant improvements [1,3,7].
For instance, BM-MSCs have the ability to differentiate into granulosa and endometrial
cells [15,84], but their long replication cycle decreases the total number of their differentiated
population [15,85]. The exact mechanism by which MSCs differentiate into target
cells, such as oocytes or supporting cells, after migrating to injured tissues, remains unclear
[1,3,7]. Thus, it has been indicated that the increase in ovarian function is mediated by
the paracrine effects of MSCs and their secretory capacity [1,3,7].
Nowadays, researchers are suggesting that the beneficial effects of MSCs in reproductive
treatments are connected to various bioactive secretory factors, including insulinlike
growth factor (IGF), vascular endothelial growth factor (VEGF), and several cytokines
[1,6,15,24,86]. Through their secretome, MSCs are able to restore tissue cellular
composition by regulating the immune response, stimulating angiogenesis, and maintaining
the viability of the microenvironment [3,24,86,87]. More specifically, MSCs, by secreting
VEGF, which binds to its receptor (VEGFR) on endothelial cells, activate the PI3K/Akt
signaling pathway; this is a critical pathway for promoting angiogenesis and enhancing cell
survival [15]. Moreover, MSCs secrete TGF-β, which engages the TGF-β receptors on target
cells. This activation leads to the phosphorylation of Smad proteins, which then translocate
to the nucleus to regulate the expression of genes involved in immune modulation and
tissue repair [88].
Moreover, the anti-apoptotic capacities of MSCs seem to be advantageous in patients
facing infertility issues due to previous or current cancer therapy treatment [8,89]. These
patients present increased expression levels of the p21 gene, which causes cell arrest in the
G1/S or G2/M phase and low expression levels of G2 cyclin, which enhances the proliferation
of granulosa cells [8,47]. Mesenchymal stem cells can increase G2 cyclin and decrease
the transcription of the p21 gene through the downregulation of p53 and Bax genes [8,47].
Also, through their secretome, they can prevent the apoptosis of ovarian follicles [11,90,91]
and contribute to resistance to oxidative stress through the upregulation of the Bcl2 antiapoptotic
protein [8,9,34]. In addition, MSCs can modulate anti-oxidative mechanisms
by increasing heme oxygenase 1 factor, which participates in inflammation [7,92,93]. In
the next section and the table below (Table 1), the multiple properties of MSCs, regarding
Life 2024, 14, 1161 6 of 21
their isolation source, are presented depending on their application in fertility-related
disorders, such as PCOS, POF, preeclampsia, Asherman syndrome, endometriosis, and
chemotherapy-induced infertility.
2.2.1. Bone Marrow MSCs
Bone marrow MSCs were first described by Owen and Friedenstein, who isolated
them from nucleated bone marrow cells in 1988 [15,94]. They are an important source of
multipotent stem cells because of their easy isolation and proliferation in vitro and their
ability to migrate effectively to damaged tissue [35]. Thus, they serve as a standard for
the comparison of MSCs derived from different sources [15,85]. They can differentiate
into chondroblasts, osteoblasts, and adipocytes [15,85], but findings have shown that they
can also differentiate into endometrial, endothelial, and granulosa cells [15,84]. Preclinical
studies in rats indicate that BM-MSCs can increase endometrial thickness and improve
receptivity and lining in rats with endometrial cavity fibrosis [17,35,95]. In addition to
that, animal studies have shown that they can induce proliferation and differentiation in
the microvascular endothelium of the endometrium through paracrine factors secretion
and they can lead to enhanced receptivity in mouse models [35,95–97]. Moreover, in
women facing infertility issues due to cancer therapy, investigators have shown that
BM-MSCs are able to restore the levels of ovarian hormones and folliculogenesis after
experimentation in a POF–chemotherapy model [10,35]. Additionally, in a clinical trial
focusing on women with POF using BM-MSCs, researchers revealed that the patients
showed hormonal improvement, resumption of menses, and an increased pregnancy
rate [8]. However, using BM-MSCs as a source of extraction remains challenging due
to their invasive isolation method and their ability to differentiate into undesirable cell
types, with increasing donor age emerging as a primary motivator in the need for extended
research [35].
2.2.2. Umbilical Cord MSCs
Umbilical cord MSCs have increased differentiation and proliferation levels, appear to
have low immunogenicity, and show an extended survival time after transplantation [35,98].
They can improve ovarian damage in infertility disorders through three major signaling
pathways: MAPK/ERK, insulin signaling, and the G-protein coupled receptor pathway
(GPCR) [14,15,99,100]. Through their regulation, UC-MSCs control proliferation, differentiation,
and cell death in eukaryotes; moreover, they can modulate cell growth and
development [101,102].
Umbilical cord MSCs are able to overturn apoptosis in ovarian cells through two
distinct ways. They can either adjust the surface epithelium of the ovaries and the tunica
albuginea, a layer of the ovarian surface, or increase the levels of TGFβ factor and CK8/18,
enhance proliferation of the cell nuclear antigen, and limit the level of Caspase 3 apoptotic
protein [14,103]. At the same time, another clinical study using UC-MSCs loaded
in collagen scaffolds demonstrated that they could restore endometrial differentiation,
vascularization, and proliferation through the enhancement of the levels of the ERα and
angiogenic factors [14,104]. Furthermore, researchers in a phase 2 clinical trial of women
with POF demonstrated that the patients appeared to have increased ovarian volume and
an increased pregnancy rate following the administration of human UC-MSCs [8]. In
addition, angiogenesis could be enhanced through the secretion of several factors from
UC-MSCs, such as the placental growth factor, VGF, TGFβ, HGF and anti-inflammatory
vascular markers, leading to decreased fibrosis and ovarian restoration [14]. Also, they
have been shown to modulate the apoptosis of ovarian cells and help in the restoration
of the ovary in both preclinical and human trials [14,49,90,105]. However, limitations of
UC-MSC application, such as their high heterogeneity, their low isolation efficiency, and
their limited collection at birth, remain challenging [35].
Life 2024, 14, 1161 7 of 21
Table 1. Overview of preclinical studies using MSCs as therapy for patients facing infertility issues.
MSCs Source Infertility
Disorder Animal Model Biological
Mechanism Treatment Outcome References
BM-MSCs PCOS Intraovarian
injection in mouse ↑ IL10
↓ Carbs inflammation,
steroidogenic gene
expression leading to
fertility recovery
[7,106]
BM-MSCs PCOS Mouse
↑ FSH, ↓ LH,
testosterone, MDA
levels
↑ Folliculogenesis,
oocyte quality,
↓ apoptosis, oxidative
stress, inflammation
[7,14,107]
BM-MSCs PCOS Intraovarian
injection in mouse
↓ CYP17A1/
DENNDIA gene
expression, BMP2,
suppression
H295R
↓ Androgen genes,
↑ apoptosis [7,14,107]
BM-MSCs Endometriosis Transplantation in
mouse
↓TNFR1
expression
↑ Folliculogenesis,
graafian follicle count,
↓ apoptosis in granulosa
cells
[7,108,109]
BM-MSCs
(CD133+) AS Rat uterus
↑ IGF1,
Thrombospondin 1
levels/IL10,
↑ FOXP3+ Treg
cells/CD163+ M2
macrophages,
↓ CD8+ cytotoxic T
cells
↑ Proliferation of
endometrial cells around
vessels leading to a
pro-regenerative
environment in which
angiogenesis, fibrosis,
receptivity, and
regeneration of the
endometrium are
controlled
[7,110,111]
BM-MSCs
(PROM1/CD133+) AS Women ↑ Er and Pr
receptors
↑ Endometrial vascular
density and improved
menstrual cycle
[35,112]
BM-MSCs Infertility after
chemotherapy Juvenile macaques -
↓ Apoptosis, fibrosis,
ovarian age, and
regeneration of blood
vessels and follicles
[14,113]
BM-MSCs POF after
chemotherapy
POF–
cyclophosphamideinduced
rabbits
↑ VEGF, estradiol,
↓ FSH, apoptotic
factor Caspase 3
↑ Ovarian function and
restored ovarian
structure
[1,15,114]
BM-MSCs Infertility after
chemotherapy
Cisplatin-induced
rat
↓ Apoptosis in
granulosa cells
Improved
perimenopause [35,115]
BM-MSCs POF Women -
Improved follicular
function, menstrual
cycle, pregnancy rate,
FSH levels, and
endometrial thickness
[8]
UC-MSCs POF Mice
Regulation of
JNK/Bcl2 pathway,
↑ HO-1, regulation
of autophagy
Ovarian restoration and
↑ of CD8/CD28 T cells [7,116]
UC-MSCs POF Mouse
Change in the ratio
Th1/Th2 cytokines,
↑ HOXA10 gene,
↑ E2, Pr, IL-4,
↓ FSH, IFNγ, IL-2
Improved implantation,
↓ apoptosis of granulosa
cells
[7,117,118]
UC-MSCs Preeclampsia
Endotoxicinduced
preeclampsia rat
↓ TNFa/IL-1β,
↑ IL-10
↓ Blood pressure, urine
protein, and white cells [7,119]
Life 2024, 14, 1161 8 of 21
Table 1. Cont.
MSCs Source Infertility
Disorder Animal Model Biological
Mechanism Treatment Outcome References
UC-MSCs PCOS DHEA-induced
mice
↓ M1 macrophages,
neutrophils,
B-lymphocytes,
TNFa, IL-1β, IFNγ,
fibrosis-related
genes (CTGF)
transcription, ↑ M2
macrophages
Regulation of
inflammation, improved
ovarian function and
recovery
[7,15,120]
UC-MSCs Preeclampsia LPS-induced rat
↑ PPARγ, laminin
receptor 1,
↓ MMP2/MMP9/ICAM1
Improved hypertension
and fetal weight [7,121]
UC-MSCs Preeclampsia AT1-AA induced
hypertension rat
Remodeling of the
spiral artery,
↓ injury in the
kidney, ↑ placental,
mesometrial
triangle HO-1
expression
↑ Pregnancy outcome,
change in the cytokine
profile of the animal
[7,122]
UC-MSCs Preeclampsia
Th1-induced
preeclampsia
mouse
↓ TNFa in uterine
and splenic
lymphocytes
↓ Blood pressure,
proteinuria,
glomerulonephritis,
↑ fetal–placental growth
[7,123]
hUC-MSCs
(Wharton jelly) Uterine scars
Transplantation
with collagen
scaffolds in rat
↑ MMP9 Endometrial renewal [35,124,125]
hUC-MSCs
(Wharton jelly) Endometriosis Endometrial cells
in vitro
↓ MMP2, MMP9,
BAC, SMAC,
survivin, Bcl2
proteins
↓ Viability, development,
invasion, migration of
the cells, ↑ their
apoptotic activity
[7,126]
hUC-MCSs AS
Injection with
collagen scaffolds
in humans
↓ ERa, Vimentin,
vonWillebrand
factor, Ki67
Recovery of uterine
adhesions, ↑ cell
proliferation,
differentiation
[15,113]
UC-MSCs Age-related
infertility
Perimenopausal
rats Cytokines release Restoration of total
follicle count [14,100]
UC-MSCs Age-related
infertility -
Phosphorylation of
FOXO1 and
FOXO3a
Primordial follicles
activation [15,127,128]
hUC-MSCs POF Women -
Improved the
development of the
number of antral
follicles, pregnancy rate,
↑ AMH, E2, FSH levels
[8]
AD-MSCs Endometriosis Rats
↓ CD68+
macrophages and
pro-inflammatory
cytokines
↓ Endometriosis-related
inflammation [7,129]
AD-MSCs POF
Injection with
collagen scaffolds
rat
-
↑ Preservation of
ADMSCs in ovaries of
the rat
[15,48]
AD-MSCs Rat ↑ VEGF ↑ Angiogenesis and
ovarian graft quality [15,130]
AD-MSCs POF
Chemotherapy
POF-induced mice,
rats
Altering gene
expression and
paracrine
cytokines secretion
Improve ovarian
function after
chemotherapy, ↑ follicle
number, oocyte number,
corpora lutea
[11,35,131]
Life 2024, 14, 1161 9 of 21
Table 1. Cont.
MSCs Source Infertility
Disorder Animal Model Biological
Mechanism Treatment Outcome References
Fetal liver MSCs POF Mouse
MT1 target,
↑ oxidate
protection,
Caspase 3, Caspase
9, Bcl2 suppression
↑ Proliferation of
granulosa cells,
anti-apoptotic effects,
↓ ovarian injury, oxidate
damage
[7,132]
Men-SCs POF Mice ↑ FGF2
Restoration of ovarian
function and structure
through ↓ fibrosis,
↓ granulosa cell
apoptosis, ↑ follicle
counts, normal sex
hormones
[7,12,15]
En-MSCs POF Mouse, rat, human
↓ Growth arrest,
GADD45B factor,
↑ CDC2 and Cyclin
B1
Restoration of ovarian
function, ↓ granulosa
cell apoptosis
[15,133–135]
Men-SCs AS Rats, rodents Regulation of PKB
signaling
↑ Angiogenesis,
immunomodulation,
rate of implanted
embryos
[7,14,136,137]
Chorionic villous
MSCs (CV-MSCs) Preeclampsia In vitro
↑ LC3BII through
JAK2/STAT3
pathway
↑ Proliferation, invasion,
autophagy of
trophoblastic cells
[7,138]
Men-SCs AS Rat
Regulation of
Wnt5a and Gdf5
factors and Hippo
pathway
↑ Endometrial growth,
improve endometrial
proliferation and
angiogenesis, ↓ fibrosis,
inflammation
[1,15,139]
Men-SCs Endometrial
damage Mouse
↑ Keratin,
vimentin, VEGF,
↓ DNA damaging
factors, PKB/AKT
signaling
modulation
↑ Rate of embryo
transplantation [14,17,35,137]
A-MSCs Age-related
infertility Mouse
Regulation of
PRKAA2/AMPK/
FOXO3/FOXO3A
pathway
↑ Ovarian function and
oocyte maturation [35,140]
A-MSCs AS Rat ↓ TNFa and IL1b,
↑ IL6 and FGFb
Ovarian restoration,
↓ inflammation [35,141]
P-MSCs - Rat
Secretion of KIT
ligand
(KITLG/SCF)
result to ↑ Lin28a,
Lhx8, Nanos3,
Nobox genes
expressions
Improve ovarian
function [35,98]
P-MSCs - Ovariectomized
rats
↑ Estrogen and
folliculogenesisrelated
genes
expression
Improve ovarian
function [15,142]
2.2.3. Adipose Tissue MSCs
Adipose tissue MSCs are easy to isolate in large quantities from adipose tissue compared
to bone marrow aspiration; the process involves a relatively simple and minimally
invasive liposuction procedure [1,35,143]. Thus, they could have potential in cell therapy
Life 2024, 14, 1161 10 of 21
in the near future. More specifically, findings have shown that their functions could be
very beneficial in Asherman syndrome treatment. Preclinical studies in Asherman syndrome
rats showed that AD-MSCs combined with estrogen therapy decrease inflammation,
improve endometrial regeneration, and enhance endometrial thickness [7,15,35,144]. In
mammals, AD-MSCs appear to have same results in combination with induction of endometrial
glands and microvessels [35,145,146]. Furthermore, in a clinical trial of women
with POF, investigators showed that the administration of AD-MSCs improved the antral
follicle diameter [8]. In addition, another study revealed that using both AD-MSCs and
BM-MSCs can improve the injured endometrium by diminishing collagen deposition and
fibrosis [7,147]. Moreover, they suggested that the intrauterine injection of AD-MSCs leads
to a thicker endometrium than intravenous injection of BM-MSCs [7,147] does, indicating
a preference for AD-MSCs application in cell therapy. However, AD-MSCs exist within a
complex environment and